The Effect of Insulin Signalling on Various Organs
Welcome to Morbus & Curis, a blog about disease and healthcare. Today’s blog post is about the effects of insulin signalling on different tissue types.
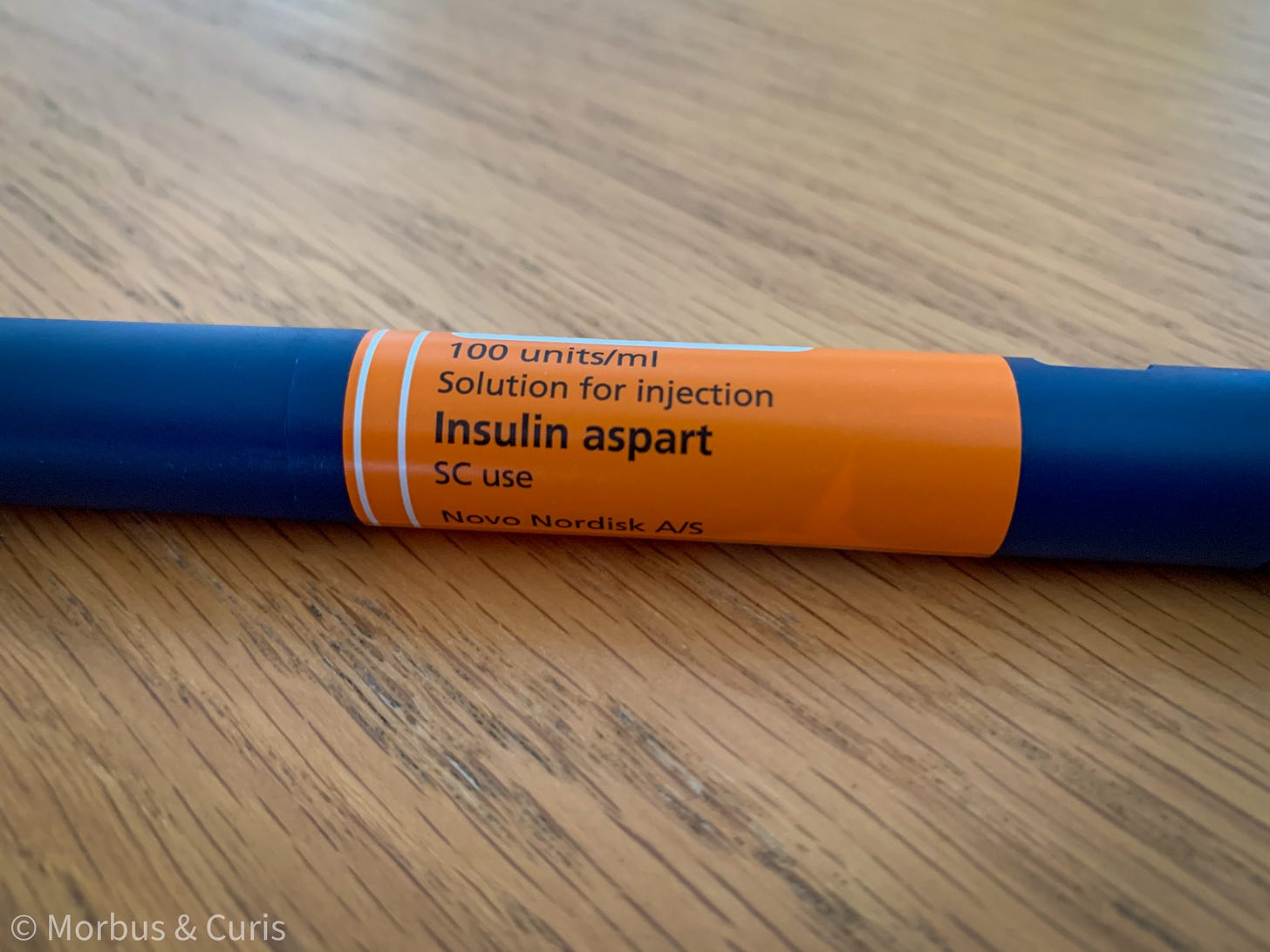
Insulin is a hormone that plays a vital role in the body’s energy homeostasis. It’s produced by pancreatic beta cells and secreted during periods of energy excess. The hormone regulates both our body’s usage and storage of glucose and fat in a range of different organs, and by doing so it helps keep our blood glucose levels stable. Consequently, a lack of insulin or an insufficient response to it leads to diseases such as type 1 and type 2 diabetes.
This article explores the different organs affected by insulin signalling and its effect on their intracellular processes.
Skeletal Muscle
Insulin signalling stimulates the uptake of glucose in skeletal muscle tissue, which serves as the main “glucose sink” of the body. Once inside skeletal muscle cells, glucose may be used for energy production or glycogen synthesis, and the rates of both of these processes are increased by insulin.
Insulin signalling also decreases the breakdown of fat and its use for energy production.
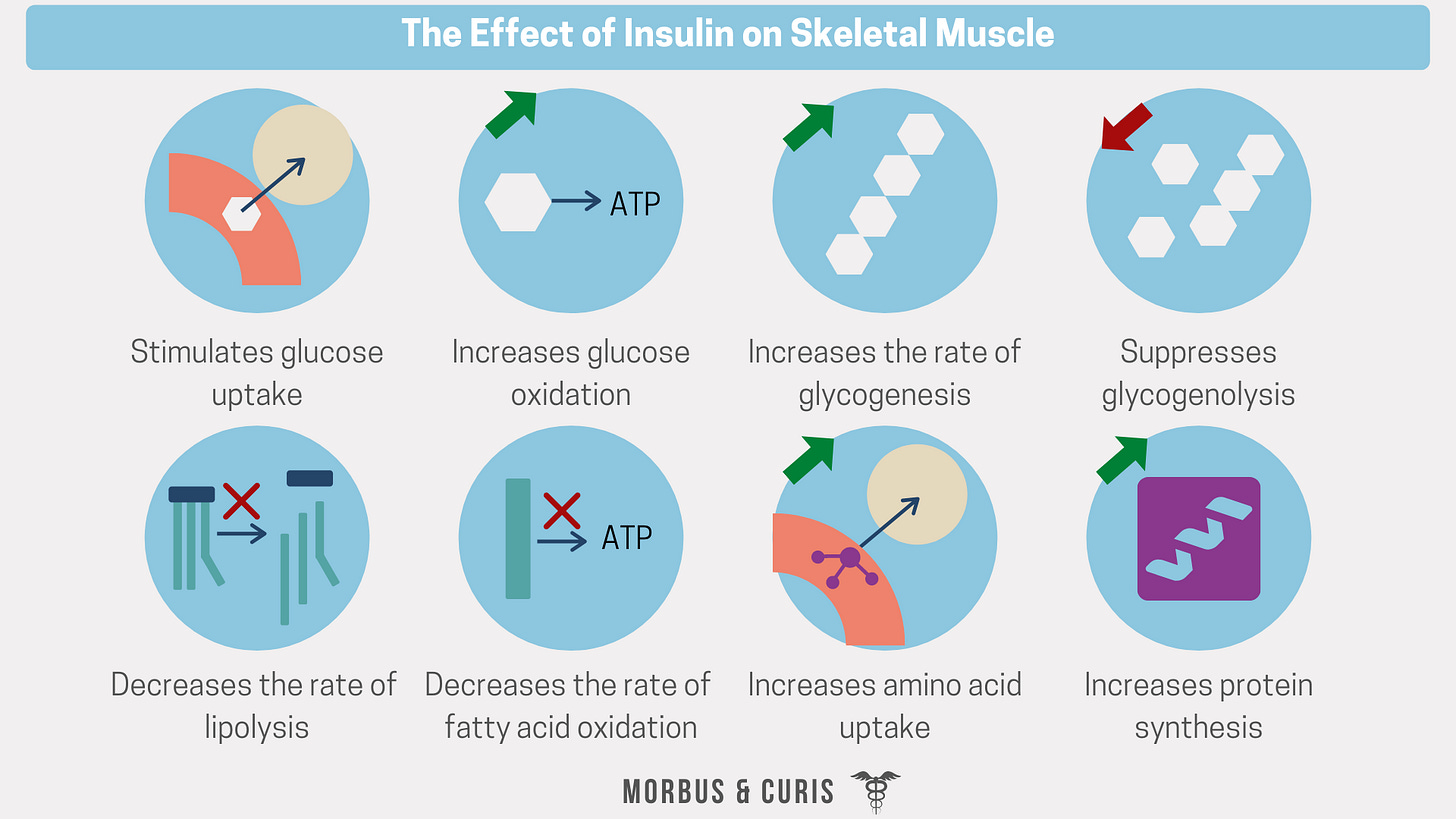
Glucose uptake and oxidation
Skeletal muscle tissue is the main site of insulin-mediated glucose uptake.
Insulin binding to the insulin receptor triggers a signalling cascade that results in the translocation of GLUT4 transporters to the cell membrane. This allows glucose uptake across the cell membrane through the transporters by facilitated diffusion.
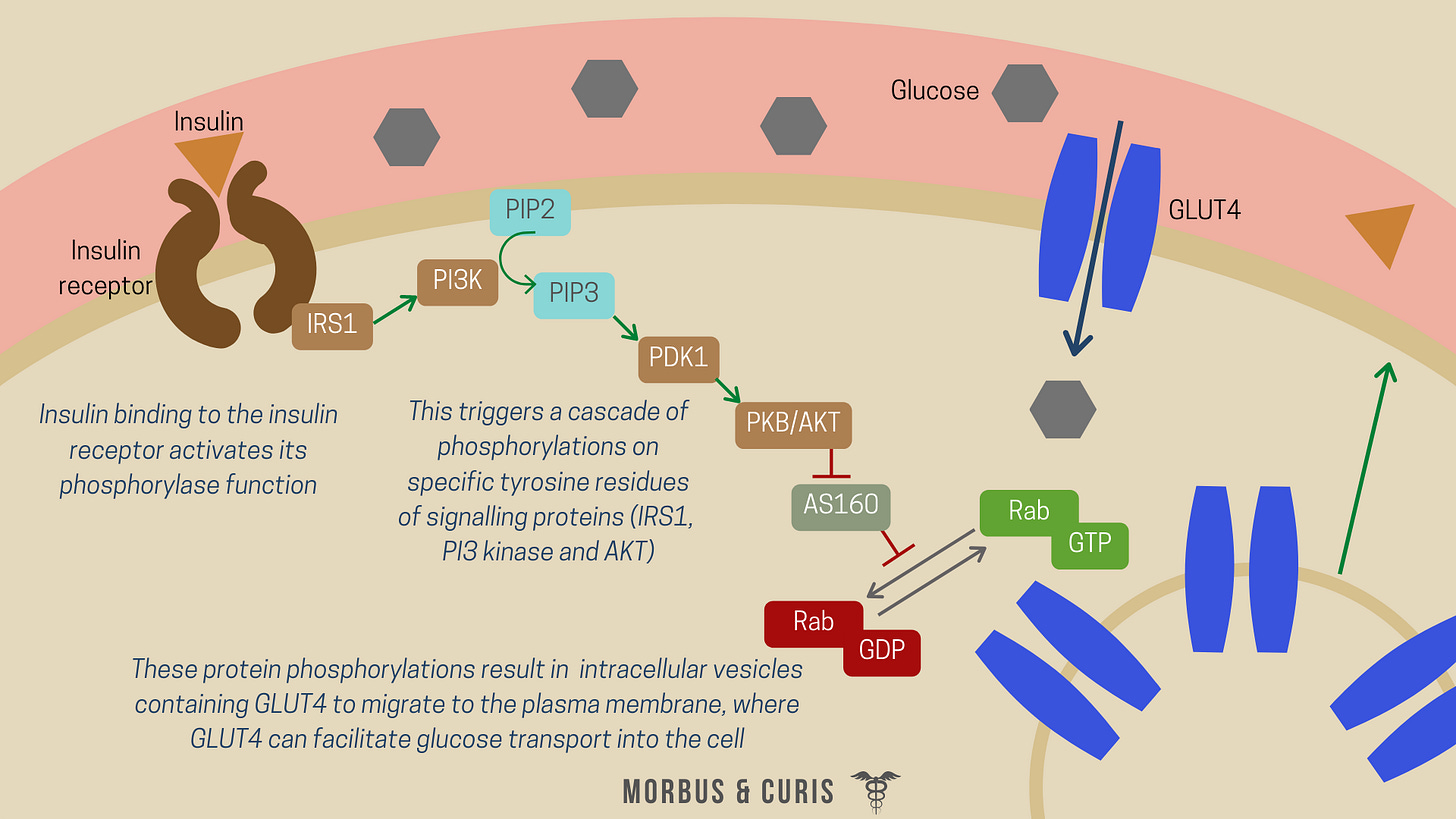
Insulin also triggers the activation of key enzymes involved in glucose metabolism, resulting in an increased rate of glucose oxidation.
Increased glycogenesis and suppressed glycogenolysis
Insulin signalling in skeletal muscle tissue also stimulates glycogenesis (glycogen synthesis). This occurs via a cascade of reactions that triggers the dephosphorylation and activation of glycogen synthase. Simultaneously, glycogenolysis (the breakdown of glycogen) is suppressed because the two processes are reciprocally regulated.
Decreased lipolysis and fatty acid oxidation
Insulin indirectly affects the rate of lipolysis and fatty acid oxidation by reducing the amount of substrate available for these processes.
Firstly, insulin signalling also stimulates the conversion of cAMP to AMP. This reduces the rate of lipolysis by reducing the amount of cAMP available as a substrate.
Secondly, as the rate of lipolysis declines, fewer fatty acids are available for fatty acid oxidation and so the rate of this process also declines.
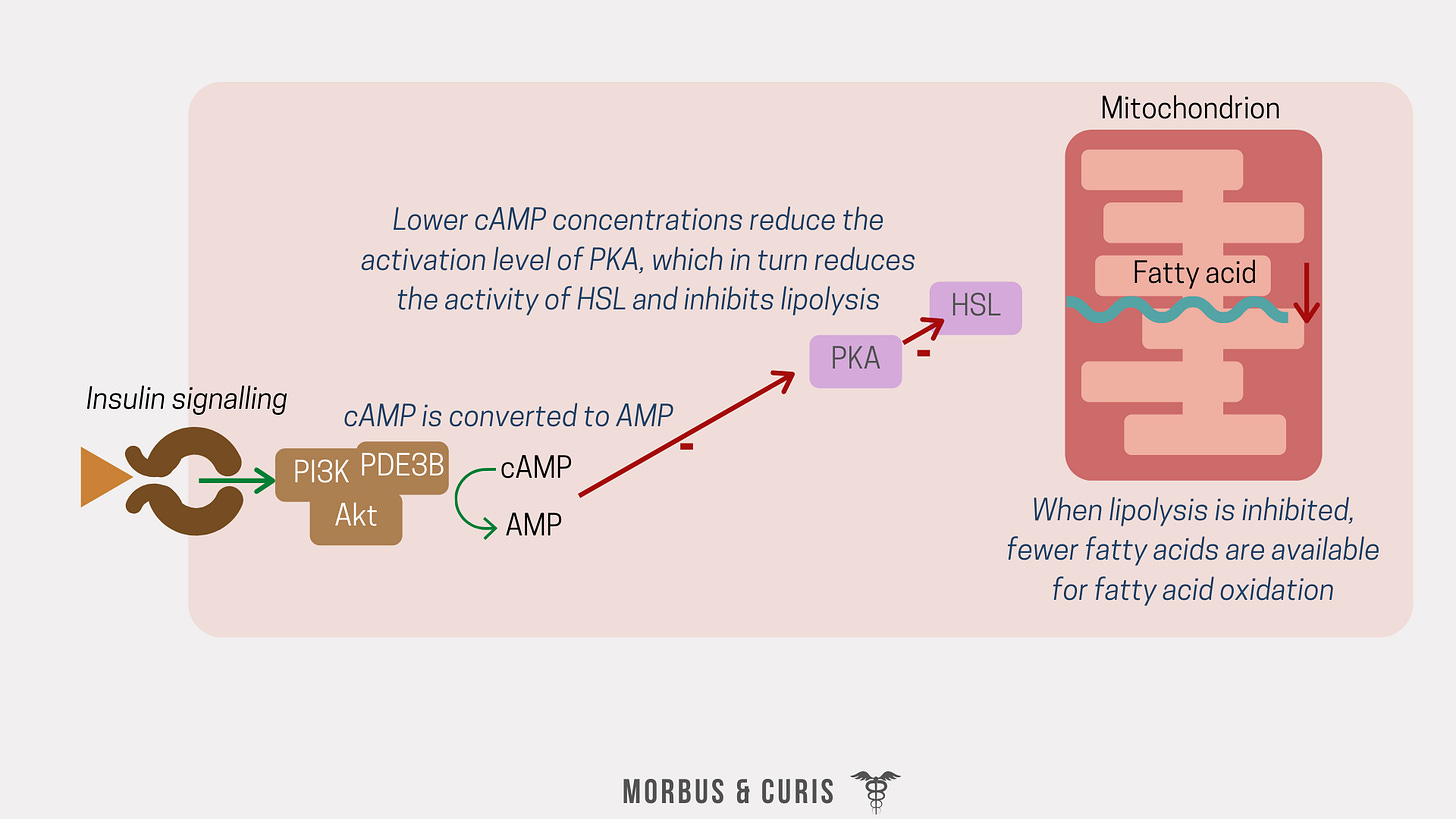
Insulin also reduces the rate of fatty acid oxidation by blocking their transport into the mitochondria, which is the site of fatty acid oxidation.
This occurs because as insulin signalling increases the rate of glycolysis, it increases the intracellular concentration of malonyl-CoA. Higher concentrations of malonyl-CoA inhibit carnitine palmitoyl-transferase, an enzyme essential for the coupling of fatty acids and carnitine before their transport into the mitochondria.
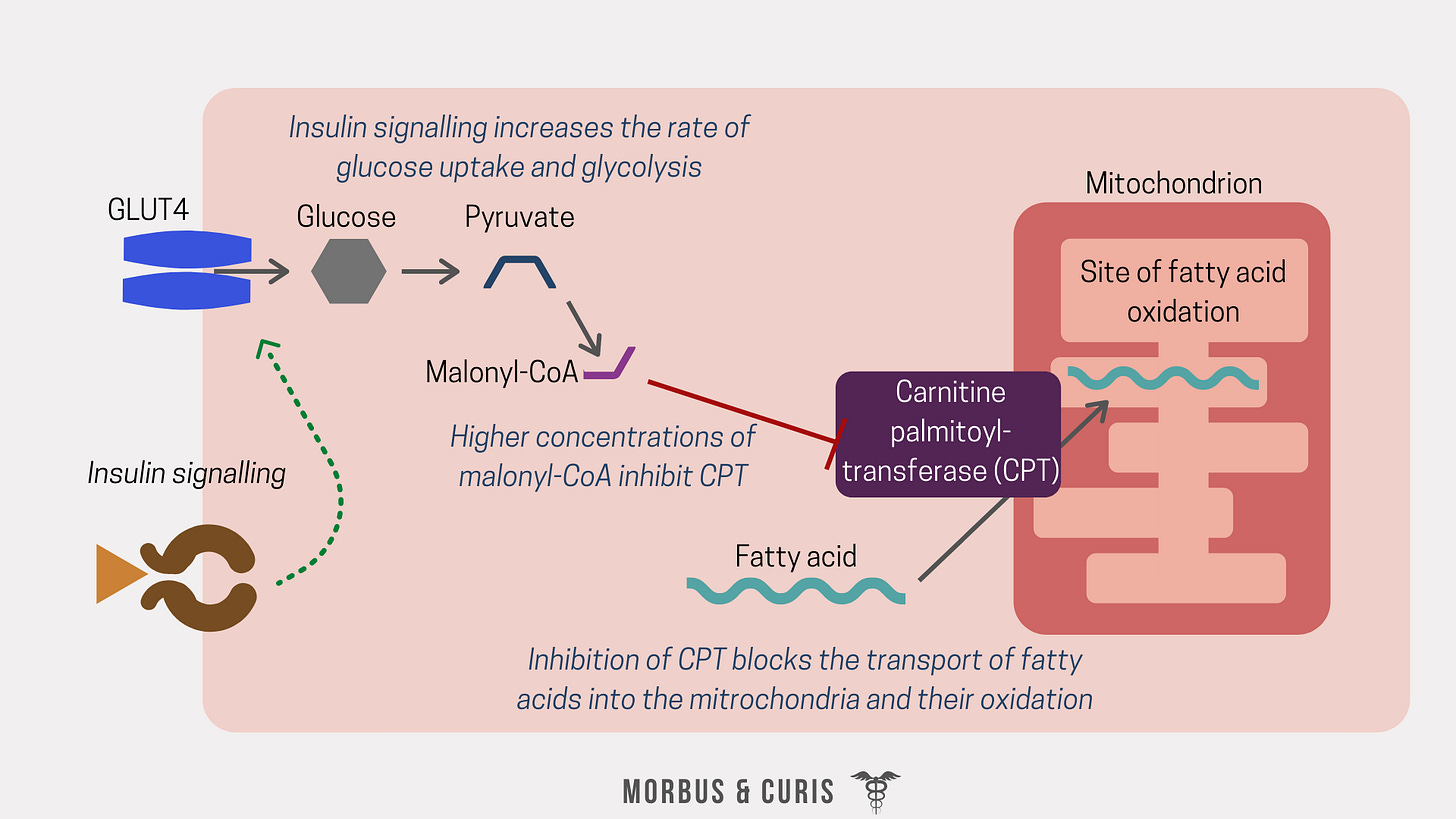
Increased amino acid uptake and protein synthesis
Insulin is believed to increase amino acid uptake and protein synthesis in skeletal muscle cells. However, how insulin achieves this is not fully understood.
Liver
The liver has a central role in energy homeostasis and insulin signalling affects a range of processes in hepatic cells. The rate of glycogen, fatty acid, triglyceride and cholesterol production are all increased, while glucose production is suppressed.
In contrast to skeletal muscle cells, glucose uptake into hepatic cells is unaffected by insulin signalling. This is because glucose enters hepatic cells via the GLUT2 transporter, which is not insulin-dependent.
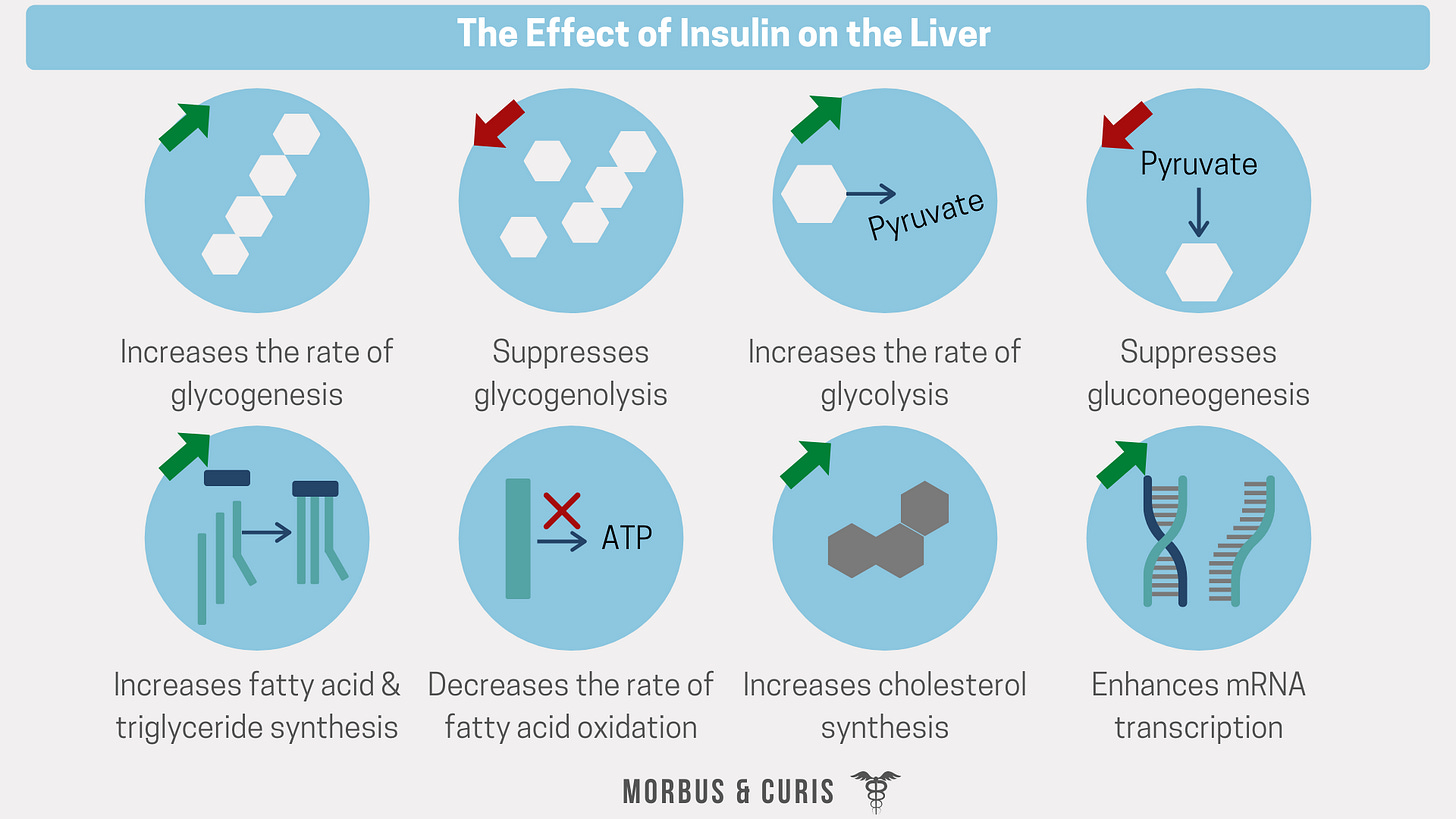
Increased glycogenesis and suppressed glycogenolysis
Insulin signalling in hepatic cells results in the storage of excess glucose as glycogen and the suppression of the breakdown of existing glycogen stores into glucose.
It achieves this by triggering a cascade of phosphorylation and dephosphorylation reactions.
Glycogenesis and glycogenolysis are reciprocally regulated via the inhibition of protein kinase A by cAMP levels. This enzyme catalyses the phosphorylation of glycogen synthase and glycogen phosphorylase, switching them to their inactive and active states respectively.
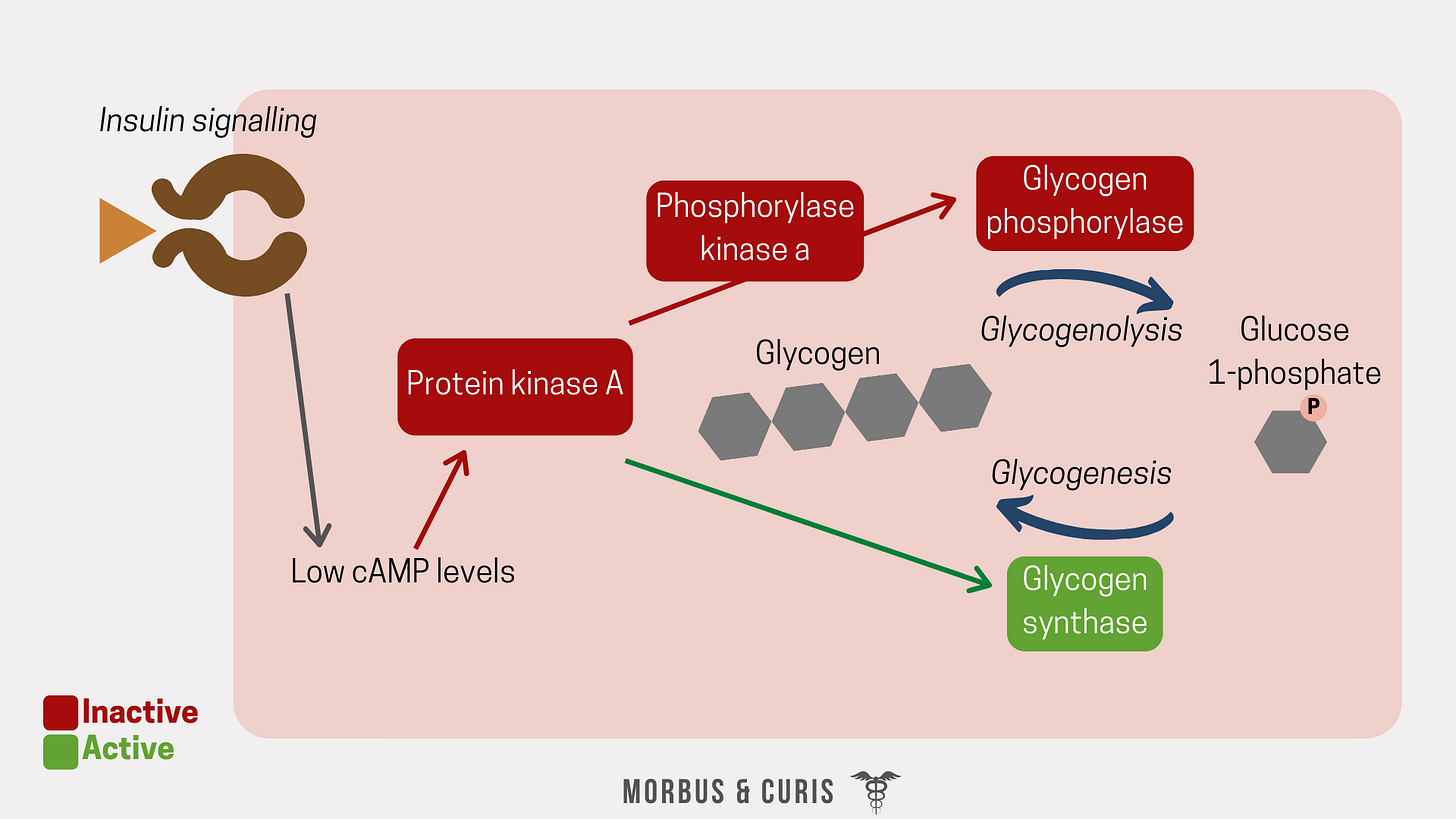
Increases glycolysis and suppresses gluconeogenesis
Insulin also stimulates glycolysis (the breakdown of glucose) and suppresses gluconeogenesis (the production of glucose).
Insulin signalling triggers the dephosphorylation of the bifunctional enzyme, 6-phosphofructo-2-kinase/fructose-2,6-bisphosphatase 1 (PFKFB1), which activates the enzyme’s kinase activity. This increases the rate of glycolysis, the products of which form substrates for energy production or fatty acid synthesis.
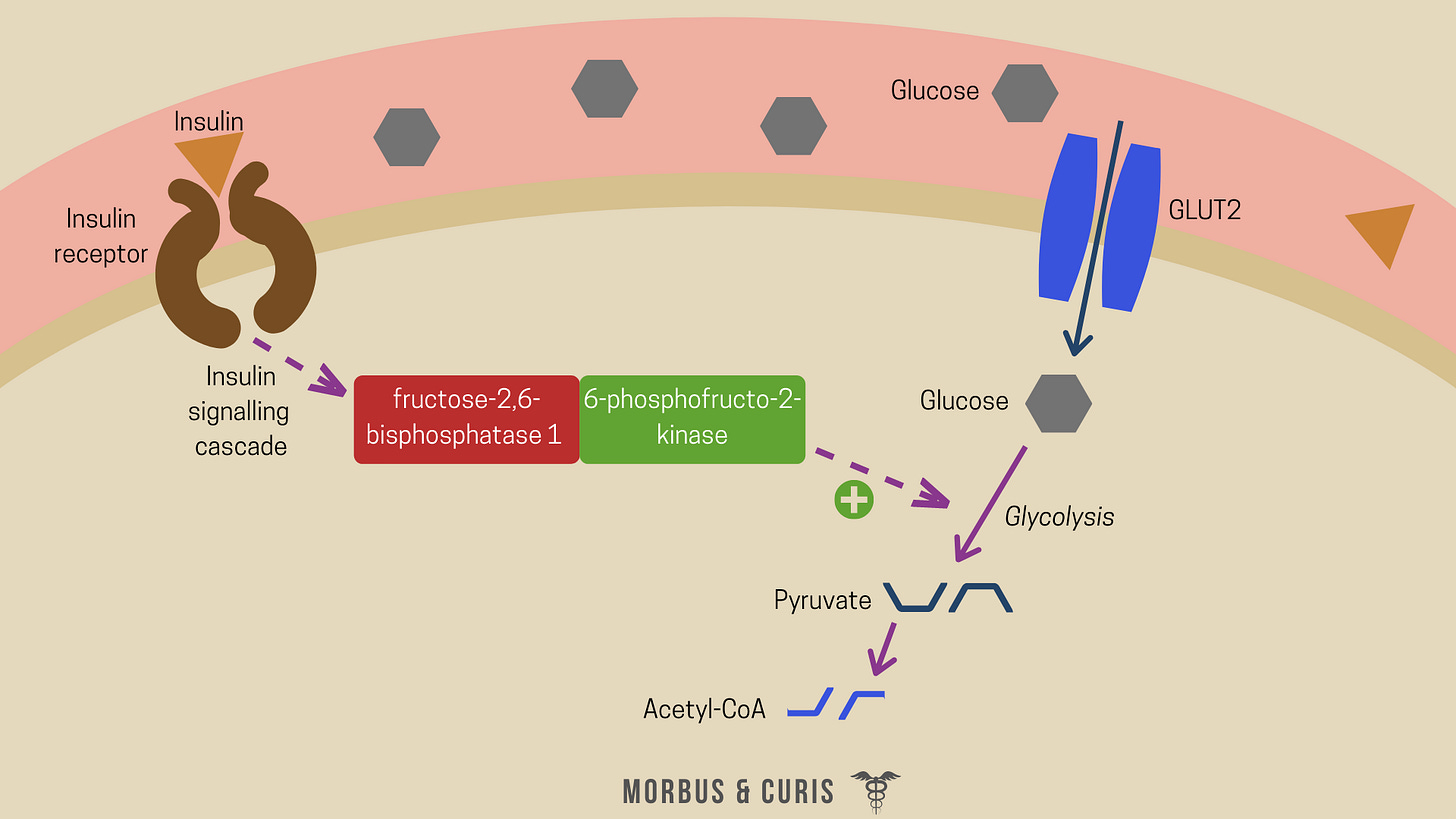
Simultaneously, the generation of new molecules of glucose from non-carbohydrate carbon substrates, such as glucogenic amino acids, glycerol and odd-chain fatty acids, is suppressed. Insulin regulates this process by negatively modulating the expression and activity of enzymes involved in gluconeogenesis, as well as those involved in the export of glucose from the liver. This includes phosphoenolpyruvate carboxykinase, PEPCK and glucose-6-phosphatase.
Increased fatty acid and triglyceride synthesis
Once the liver has reached its glycogen storage capacity, the conversion of glucose into fatty acids and the synthesis of triglycerides is stimulated.
Insulin accelerates these processes by triggering the activation of:
Pyruvate dehydrogenase - an enzyme involved in the conversion of pyruvate to acetyl Co-A, which is a substrate for fatty acid synthesis.
Protein phosphatase 2A - an enzyme that dephosphorylates and activates acetyl-CoA carboxylase, an enzyme involved in fatty acid synthesis.
Since the liver cannot safely store fat, newly formed triglycerides are packaged into lipoproteins (VLDL) that can enter the circulation and be sequestered by adipose tissue.
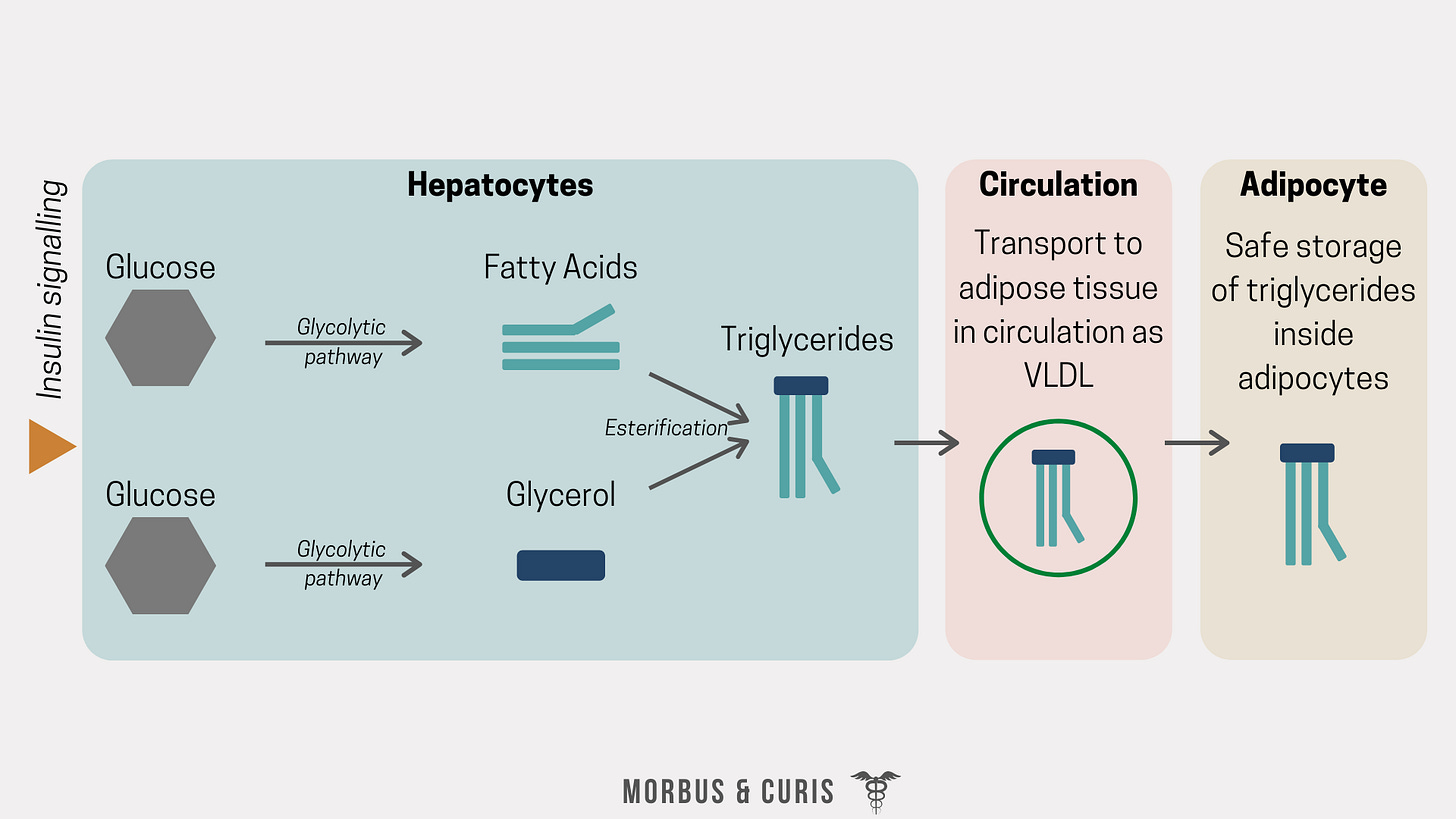
Decreased fatty acid oxidation
Similar to skeletal muscle, insulin decreases the rate of fatty acid oxidation in the liver by triggering a cascade of reactions that result in the inhibition of carnitine palmitoyl-transferase. Again this blocks the transport of fatty acids into the mitochondria, where fatty acid oxidation takes place.
Increased cholesterol synthesis
Cholesterol synthesis is increased by the dephosphorylation and activation of HMG Co-A reductase (HMGR). Insulin is believed to play a role in triggering this by activating the phosphatase that removes phosphates from HMGR, which converts it to its active form.
Additionally, by increasing the rate of glycolysis, insulin signalling increases the amount of acetyl-CoA available for cholesterol synthesis.
Enhanced mRNA transcription
Insulin enhances the transcription of mRNA for glucokinase, pyruvate kinase and fatty acid synthase in hepatic tissue. The activity of all of these enzymes is increased during times of energy excess.
Adipose tissue
Insulin signalling also stimulates the uptake of circulating glucose and triglycerides in adipose tissue. It also stimulates the production of triglycerides from glucose.
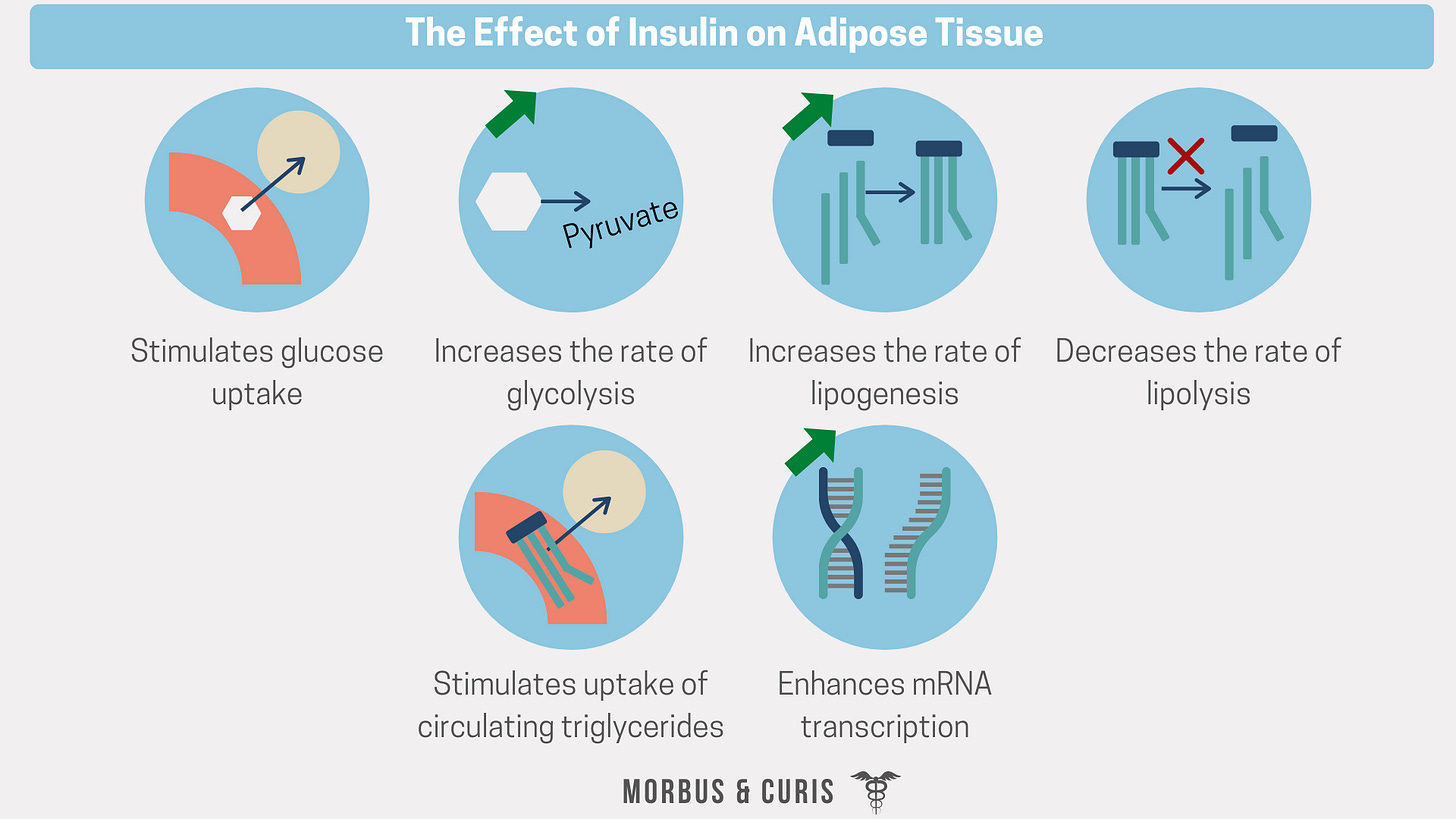
Glucose uptake
Similar to skeletal muscle tissue, facilitated diffusion of glucose across the cell membrane is mediated by GLUT4 transporters, and the translocation of those transporters to the cell membrane is insulin-dependent.
Once inside the adipocyte, glucose can be used as a substrate for energy production or broken down into substrates that can be used during triglyceride synthesis.
Increased triglyceride uptake
Insulin signalling also stimulates the uptake of circulating triglycerides into adipocytes.
Triglycerides are esters formed by the condensation of glycerol and three fatty acids. They are produced in the liver during periods of energy excess and carried in the circulation in very-low-density lipoproteins (VLDL). While VLDLs cannot be transported across cell membranes, glycerol and fatty acids can.
Insulin stimulates the uptake of triglycerides carried in VLDLs in two ways:
It activates a lipoprotein lipase located on the cell surface of adipocytes that hydrolyses circulating lipoprotein triglycerides into free fatty acids and glycerol, which can then be transported across the cell membrane.
Increasing the expression of fatty acid-binding proteins that facilitate the transport of fatty acids across the cell membrane.
Once free fatty acids and glycerol have been transported into an adipocyte, triglycerides can be reformed then stored until required during times of energy deficit.
Increased glycolysis and lipogenesis
Insulin also stimulates the synthesis of triglycerides from excess glucose broken down via the glycolytic pathway. This process creates various different molecules, including pyruvate and dihydroxyacetone phosphate.
Pyruvate is then converted into acetyl CoA molecules, which are condensed together to form fatty acids, while dihydroxyacetone phosphate is converted into glycerol. Subsequently, fatty acids and glycerol can be combined to form triglycerides.
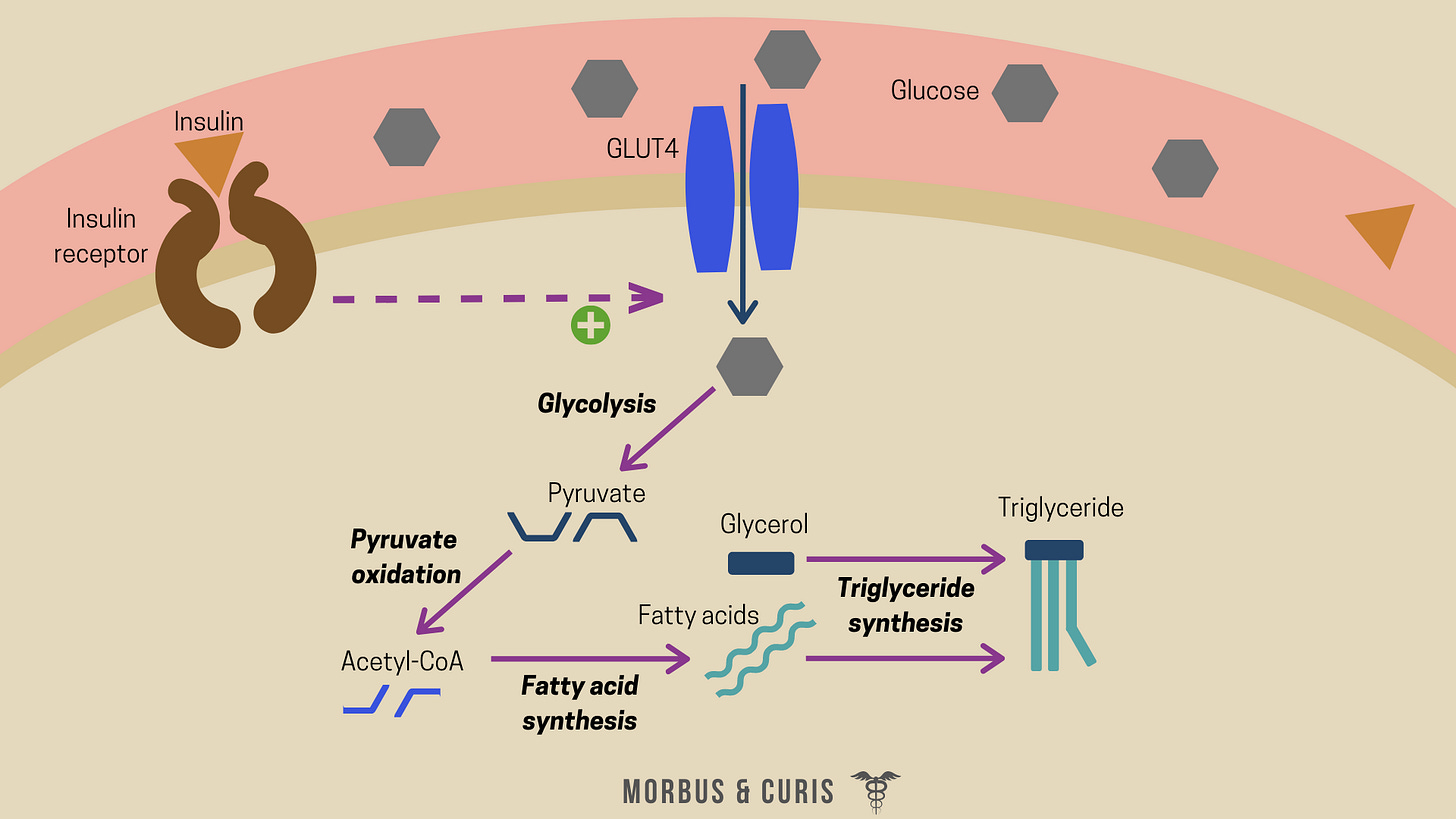
Decreased lipolysis
Insulin decreases the rate lipolysis of stored triglycerides by inhibiting the action of the enzyme Hormone Sensitive Lipase (HSL), which hydrolyses triglycerides into glycerol and fatty acids. This reduces the amount of free fatty acids (FFAs) that can leave the adipose tissue and enter the circulation, thereby decreasing plasma levels of fatty acids during periods of energy excess.
Enhanced mRNA transcription
Insulin enhances the transcription of mRNA for pyruvate carboxylase, an enzyme involved in the glycolytic pathway.
Hypothalamus
Insulin signalling also occurs in the hypothalamus, where it has been shown to suppress food intake, reduce glucose production in the liver and increase lipid production in adipose cells.
Insulin’s effect on both satiety and glucose metabolism occurs via a common pathway of phosphorylation from the insulin receptor to PI 3-kinase.
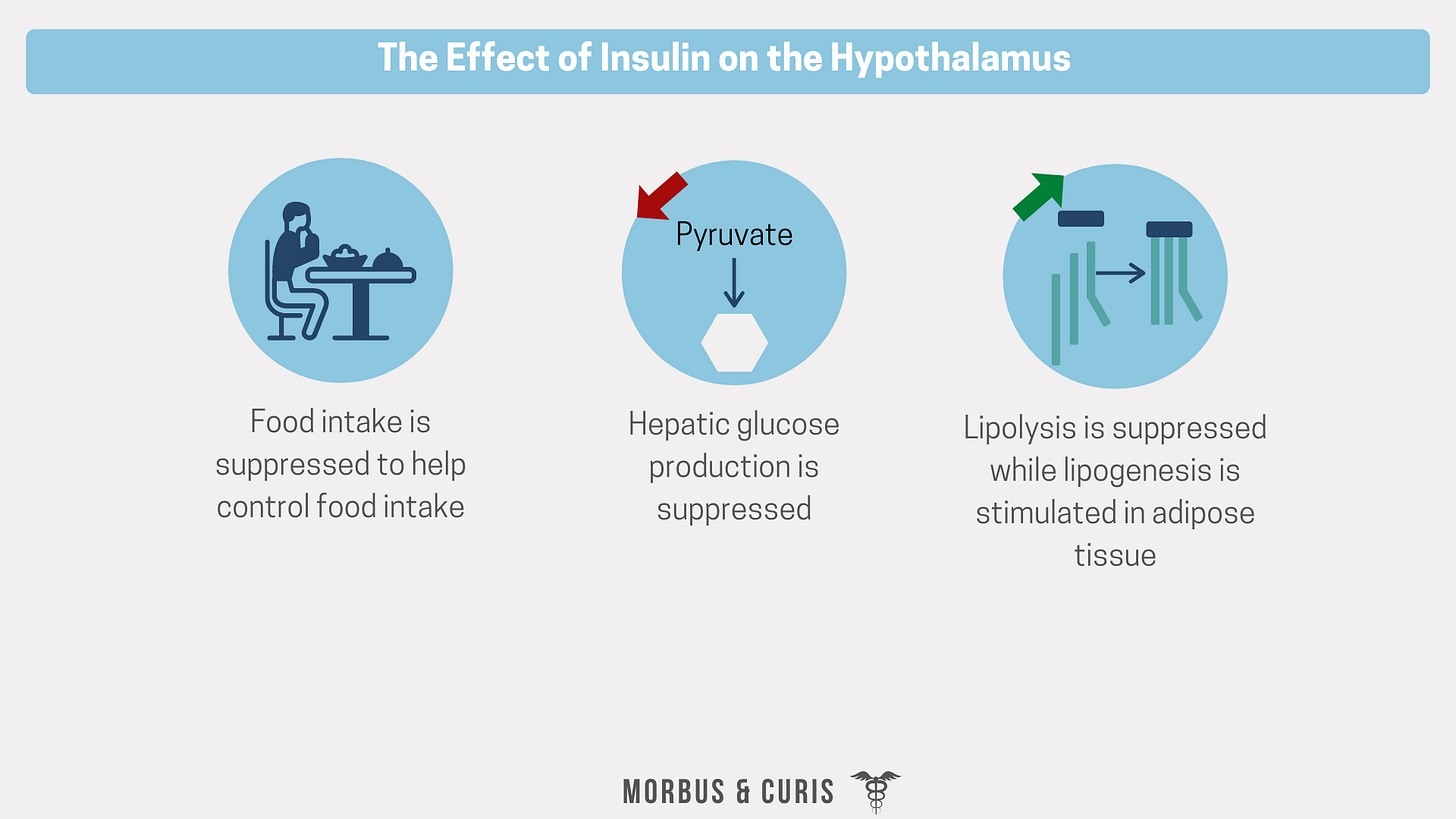
Summary
Insulin has a key role in maintaining stable blood glucose levels by controlling the rate of uptake, oxidation, production, storage and breakdown of various energy substrates. Its primary function is to reduce blood glucose levels by increasing the uptake of glucose in skeletal muscle, hepatic and adipose tissue. It also instructs cells to preferentially oxidise carbohydrates and stimulates the synthesis and storage of triglycerides.
Accordingly, insulin has a vital role in cellular energy supply and macronutrient balance during periods of energy excess.
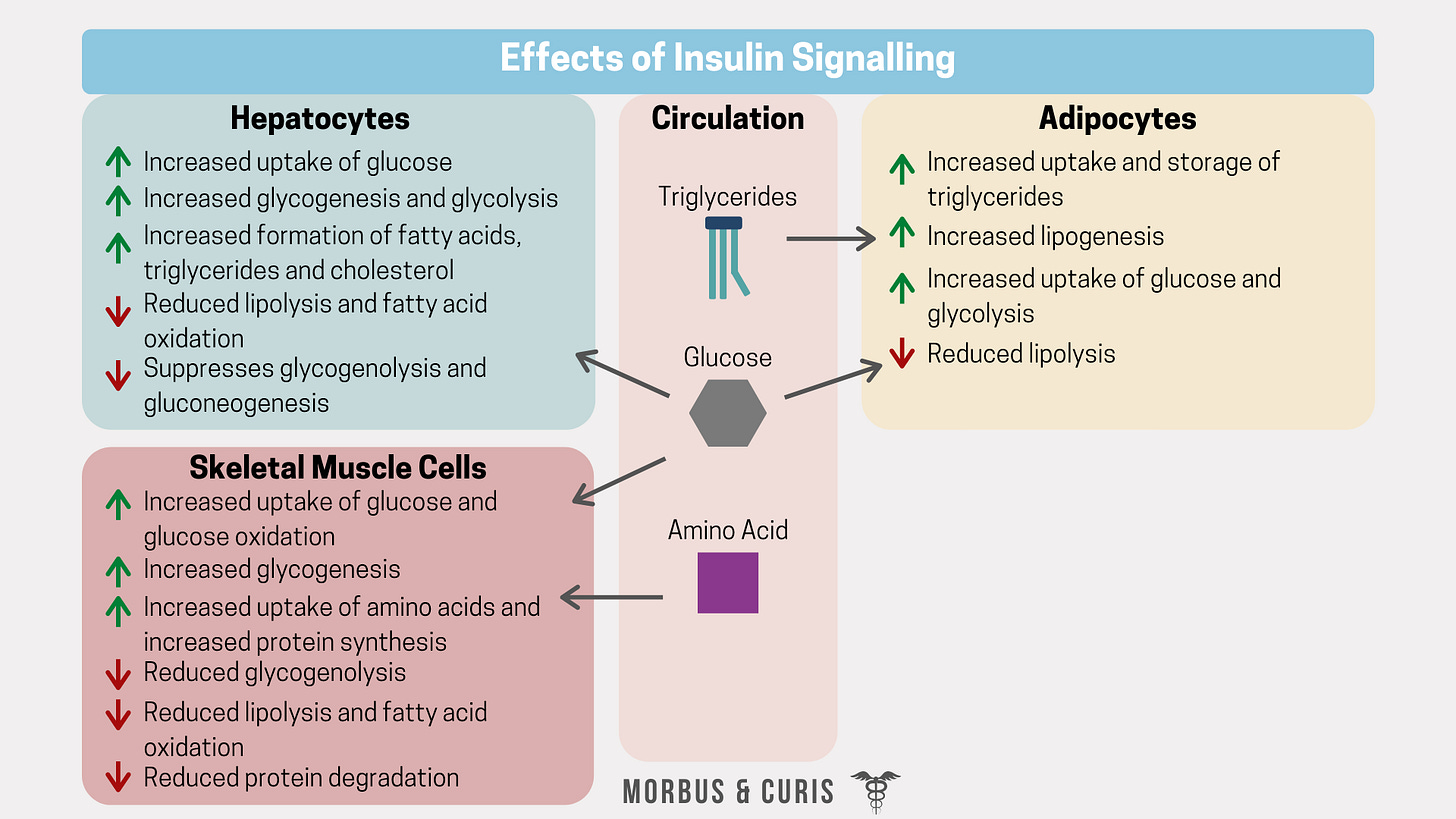
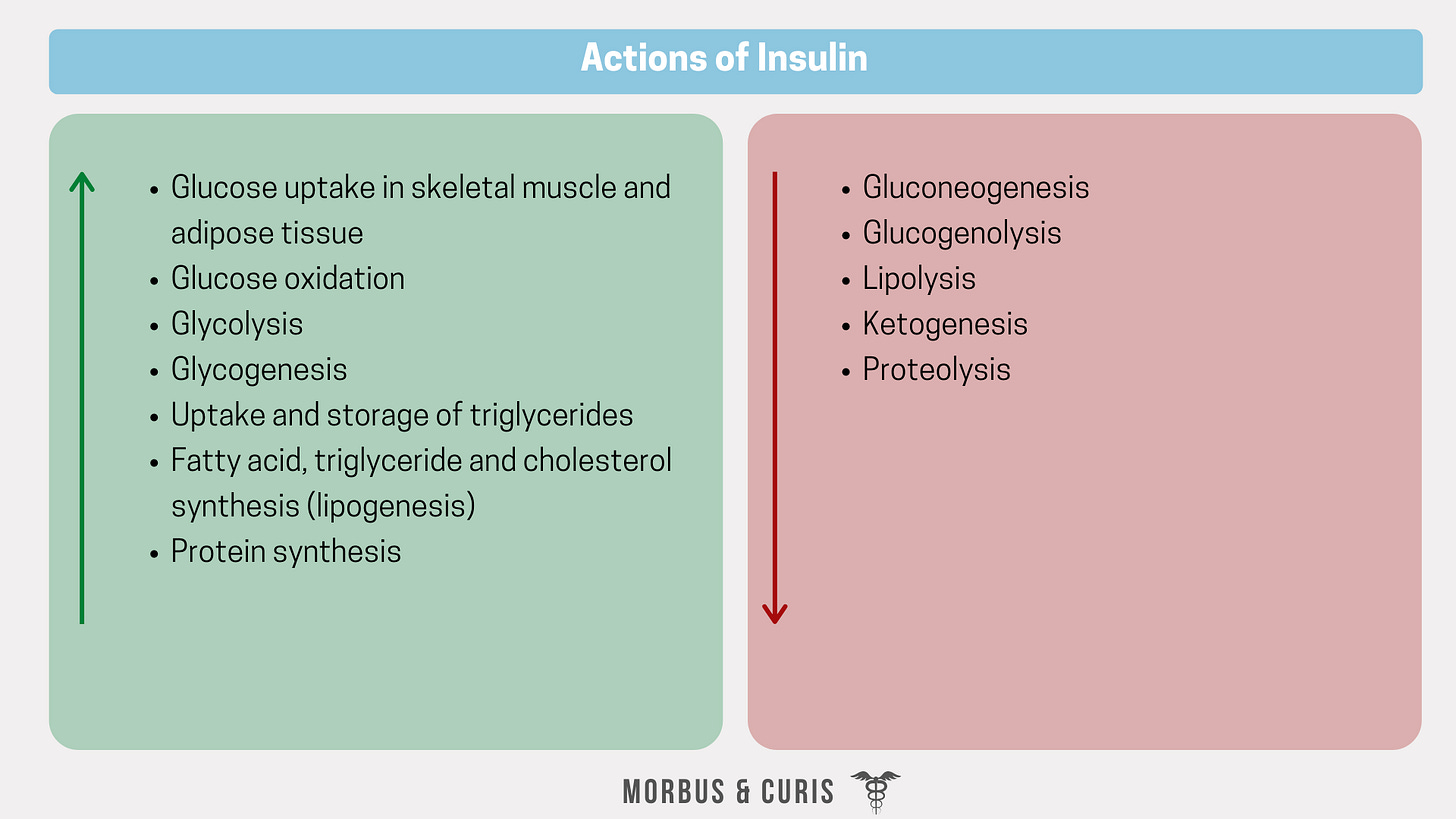
Sources
Insulin and Insulin Resistance
Insulin effects in muscle and adipose tissue
Glycogen Breakdown and Synthesis Are Reciprocally Regulated
Insulin signalling mechanisms for triacylglycerol storage
If you found this blog post helpful why not consider subscribing.